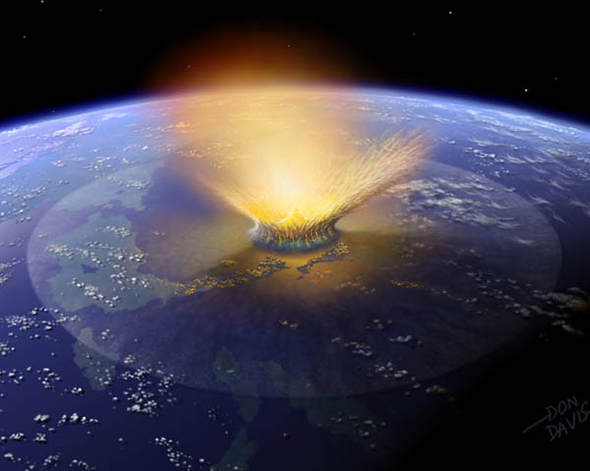
Life on Earth may have started with a bang.
There aren't a lot of individual experiments that have ended up being staples of high school textbooks, but Stanley Miller and Harold Urey did one of them. Miller and Urey are the people who sealed up a mixture of gases meant to model the Earth's early atmosphere and jolted the gas with some sparks. What emerged was a complex mix of chemicals that included amino acids, the building blocks of proteins.
It was a seminal experiment in that it gave researchers one of the first avenues to approach the origin of life experimentally, but its relevance to the actual origin of life has faded as the research it inspired began to refine our ideas. A French-Czech team of researchers decided to give it another look, using a source of energy that Miller and Urey hadn't considered: the impact of a body arriving from space. The result? The production of all four of the bases found in RNA, a close chemical cousin to DNA and equally essential to life.
Conceptual shifts
There are two reasons that the Miller-Urey experiment gradually fell out of favor. The first is conceptual. At the time, people focused on life's dizzying web of chemical reactions, almost all of which are catalyzed by proteins, so it was hard to envision life without proteins. The formation of amino acids could enable the formation of proteins and thus seemed to provide an obvious route to a primitive biochemistry. Genetic material could be added later.
What Miller and Urey didn't know, and what we wouldn't find out for decades, is that proteins don't have a monopoly on cells' catalytic chemistry. We have found that a number of key reactions in the cell can be catalyzed by RNA; both RNA and DNA have since been shown to be able to participate in all sorts of interesting chemistry. Those discoveries helped usher in the RNA world hypothesis, which posited that biochemistry and genetics got their start simultaneously in the form of catalytic RNA molecules.
Amino acids might be useful co-factors to RNA or byproducts of the reaction, but they weren't central to the start of life. The challenge wasn't showing how amino acids could form under simple reaction conditions; instead, the focus shifted away from Miller-Urey and onto ways of forming the chemical bases that are the building blocks of RNA.
In addition to conceptual problems, the original experiment ran into practical ones. Miller and Urey assumed what's called a "reducing" atmosphere, unlike today's oxidizing one. In practical terms, this means something akin to that of Saturn's moon Titan, where the major components of our atmosphere are linked to hydrogen atoms—so ammonia instead of molecular nitrogen, water and methane instead of carbon dioxide, and no oxygen on its own. Ideas have shifted on that, and evidence suggests that the early Earth atmosphere is likely to have been neutral, somewhere between reducing and oxidizing.
Revisitation
The people behind the new research argue that current evidence suggests that more than 3.6 billion years ago, the atmosphere was a bit on the reducing side of neutral. In any case, it's clear that the early Earth was being hit regularly by things like comets, which carry a large payload of reduced chemicals. So while a Miller-Urey style atmosphere may not have dominated, they argue it would have been relevant.
They redid the Miller-Urey experiments, but this time they looked for the presence of a different chemical: formamide, which has one atom each of carbon, nitrogen, and oxygen, with three hydrogens thrown in. While simple, it's an important chemical building block. Experiments by other researchers have shown that under the right conditions, formamide can react with itself to form all of the four RNA bases.
Miller and Urey wouldn't have known how significant of a finding it was, but it definitely showed up in the new work. It didn't go on to form any of the RNA bases, but that was expected, as these reactions need specific catalysts or radiation at UV wavelengths. The authors, however, noted that researchers had described another option for catalyzing the formamide reactions: shock waves, such as those from extraterrestrial impacts.
Conveniently, the team had access to the Prague Asterix Laser System, which can generate Terawatt-sized pulses. They used the system to mimic the sorts of shock waves that an impact would produce.
In the shock wave reactions, formamide was also formed. But it went on to form all four of the bases found in RNA. Most of them were just present in small quantities, some barely above the detection limits. Of course, the shock waves of a real impact would be larger and more sustained, so the quantities should scale considerably.
The new work doesn't deal with the debate about how likely this sort of atmosphere would be on the early Earth. But the key takeaway is that the research adds to the multiple plausible routes we have for producing the building blocks of RNA. It also has relevance for the prospects of finding life elsewhere. Even if the early Earth didn't quite have a Miller-Urey style atmosphere, with billions of exoplanets to choose from, chances are a number of them did.
PNAS, 2017. DOI: 10.1073/pnas.1700010114 (About DOIs).